1. Introduction
Iron oxide nanoparticles are located on the earth’s exterior and are mostly noted as magnetite (Fe3O4) and maghemite (Fe2O3). Nanocrystals are the conformation of iron oxide NPs utilized in energy, medical, and environmental applications. These nanocrystals are synthesized and originate from volcanic eruptions, traffic and industry wastages, and fires [
1-
9].
More than that, iron oxide NPs are incrementally utilized in sewage clean-up [
8,
10-
12]. The cause of surrounded magnetite nanoparticles in a zeolite host was the effortless manipulation of large particles and employing them for attaching and eliminating ionic pollutants in the role of ion exchangers [
13] or declining the bioavailability of heavy metals in the polluted ground [
14]. Moreover, using as an adsorbent factor in pharmaceutical outcomes is the utilization of iron oxide NPs merged in zeolite [
15]. In the last twenty years, Magnetic NPs (MNPs) have been considered and are being studied for employment in tumor therapy and imaging [
16]. Also, alongside their utilization in the role of MRI contrast factors in the investigations of targeting tumor cells with biomolecules like anticancer drugs, antibodies, and siRNA, the utility of MNPs is evaluated [
17-
20]. The desirable toxicity characteristics and competency of crossing the blood-brain barrier (BBB) cause iron oxide-based MNPs to be an appealing Nano platform for malignant brain tumor therapy [
21]. US FDA-approved formulations of iron oxide-based MNP are in clinical employ for abdominal imaging [
22], and the investigations of their usage in brain tumors continue to be researched. iron oxide-based MNPs have indicated enormous capacity as T1 or T2 contrast factors in MRI imaging [
23, 24] with superparamagnetic iron oxide-based NPs as the most commonly explored type of MRI contrast factors [
25].
The probability of applying ingrained forms of nanoparticle ingredients has been presented. Nanoparticles have special and unexampled physiochemical characteristics due to their tiny size (<100 nm), high surface-to-mass ratio, singular quantum features [
26], and, therefore, exclusive biological attributes. Crossing through cellular and nuclear membranes is a special feature of smaller nanoparticles, and they can perforate cells and intracellular constructions and aim at delineated points within the body [
27].
The conception period corresponds to a critical moment because of restructuration procedure incidence at both molecular and cytological levels. Therefore, organism formation is sensitive to any substance that may cause modifications. In addition, because of the uttermost decline in their sizes, NPs can perforate to the biological barriers and cellular membranes, affecting the viability of the reproductive cells and embryo development [
28].
Although documentation of the outcomes of inserting Platinum nanoparticles into an organism is not yet well, even extremely tiny amounts of nanoparticles or unbound ions may defeat the BBB and influence the BBB and brain performance after penetrating the brain tissue. Also, some reports indicated that cell death by apoptosis in the brain tissue may be caused by diverse types of nanoparticles in different sizes (20 to 300 nm) and constructed from various materials [
29].
The hypothesis of this experimental study was that iron oxide NPs may affect the growth and development of embryos and can cross the BBB and cause morphological alterations in brain tissue. Consequently, the objective of this preliminary study was to investigate the effects of iron oxide NPs on embryo growth and development with an emphasis on brain morphology, concerning their potential applicability in brain cancer therapy.
2. Materials and Methods
For this experimental study, in a completely randomized design template, 160 fertile eggs of the Ross 308 broiler strains at 49 weeks of age with 65 gr weight mean were bought out from Chicken Complex Tabriz (Ilkhchi) Motherhood. After recognizing the air sac with candling, Fertile eggs were divided into 8 groups (n=20). The eggs were incubated in a setter at 37.4°C and 71-75% relative humidity and in a hatcher (three last days) at 37.1°C and 75-81% relative humidity. Group 1 (control) received 0.3 mL serum normal. Groups 2, 3, and 4 received iron oxide NPs as maghemite (Fe2O3) with doses of 100, 250, and 500 ppm, respectively. Groups 5, 6, 7, and 8 received iron oxide NPs as magnetite (Fe3O4) with doses of 100, 250, 500, and 2500 ppm, respectively. All injections were carried out on the first incubation day in 0.3 mL of volume into the air sac with a 22-gauge needle. Doses of Nanoparticles were obtained in a pilot study. All substances were sterilized in an autoclave. Iron oxide NPs were prepared with 20-40 nanometers in size from Pishgaman Nano Mavad, Iran. Twenty days after injections, 20-day-old embryos were extracted from the eggs by the fraction of the calcic membrane. On death day, chicken, brain, liver, heart, bursa of Fabricius, spleen weights, and teratogenic samples were recorded, and brain tissues from all chickens were collected for histological preparations. The brain tissues were fixed in a 10% neutral formalin solution and routinely processed for light microscopy and embedded in paraffin. Thin sections (5 μm) of the brain were cut using a microtome and stained with hematoxylin-eosin. After staining, the tissue sections were dehydrated in alcohol, cleared in xylene, and mounted in a resinous mountant (Canada Balsam). Histological analysis was performed under a light microscope (Diaphot, Nikon, Tokyo, Japan) equipped with a camera and the Axiovision software, version 4.8 (Carl Zeiss, Oberkochen, Germany) regarding the changes in different groups compared to the control group. The images were processed using Adobe Photoshop CS, version 8.0 (Adobe system, San Jose, CA).
Statistical analysis
All data were expressed as the Mean±SD. The variables were analyzed by one-way ANOVA followed by Tukey’s test for post hoc comparisons using SPSS software, version 18 (SPSS Inc, Chicago, Illinois, USA). The statistical significance level was set at P<0.05.
3. Results
Anatomical and embryological findings
Evaluation of teratogenic indices of iron oxide NPs as retaining out of the heart from the thorax, exposing of the digestive tract, and defects in the formation of the abdominal cavity wall indicated that in experimental groups 2-8, teratogenous embryos were significantly increased in comparison with the control group (P<0.05). The most teratogenous embryos were observed in groups magnetite 100 and 500 and maghemite 500. Teratogenous embryos were lower in magnetite 250 and maghemite 250 groups (
Figure 1A).
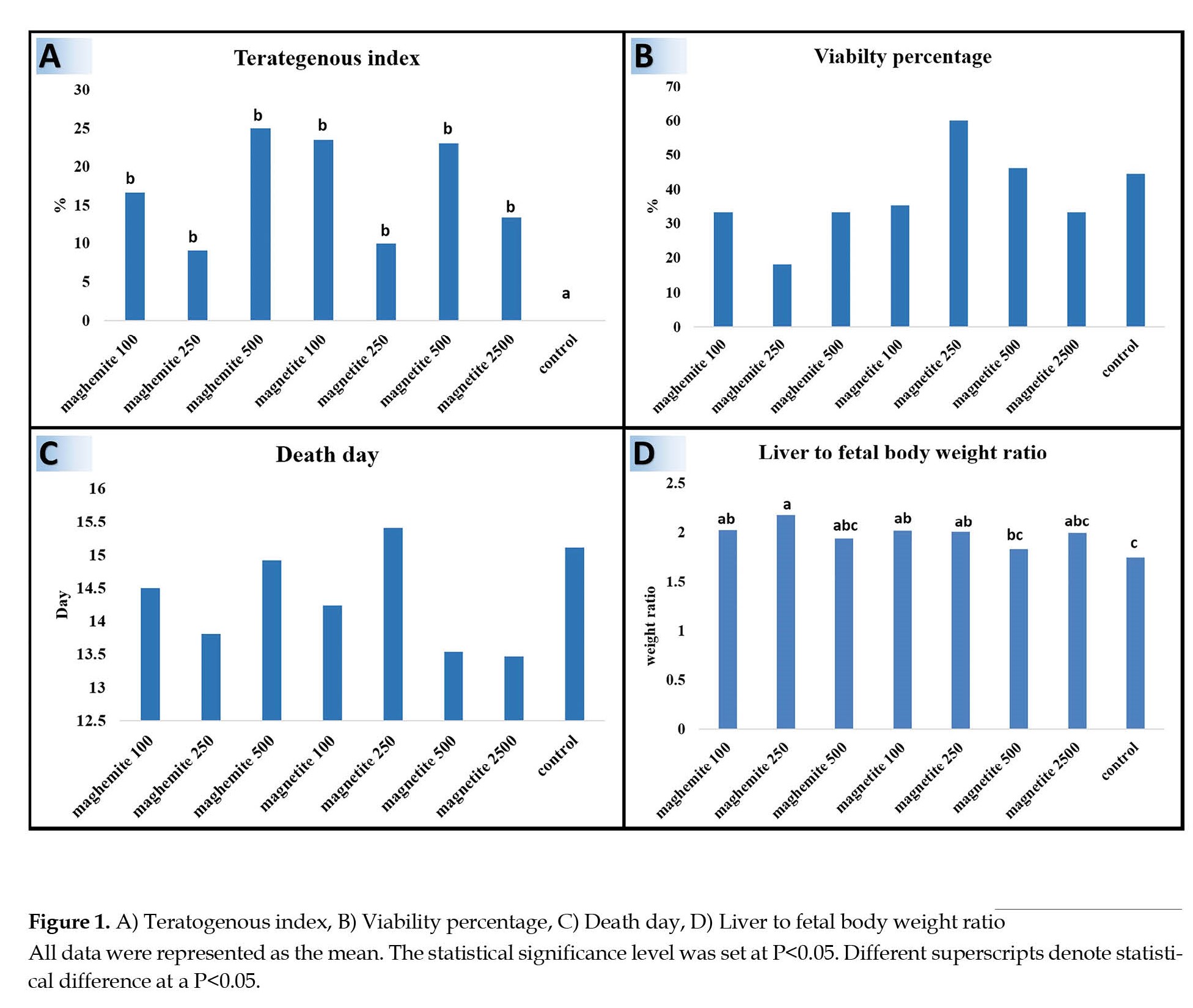
Investigation of 20 days’ age embryos viability percentage and death day of embryos showed no significant difference between experimental and control groups (P>0.05). The highest viability percentage was in group maghemite 250 and the lowest one was magnetite 250 (
Figure 1B). In groups magnetite 500 and 2500, the embryos were dead in the first days of the fetus (
Figure 1C).
The liver-to-fetal body weight ratio assessment revealed that the liver in the control group was smaller than in other groups. Also, the mentioned ratio in the control group was significantly low compared to magnetite 100 and 250 and maghemite 100 and 250 groups (P<0.05). The highest liver-to-body weight ratio was observed in group maghemite 250, which showed a significant increase compared to control and magnetite 500 groups (P<0.05) (
Figure 1D).
The spleen to fetal body weight ratio in experimental groups was decreased compared to control groups, but, statistically, there was a significant difference between control and maghemite 250 and 500 groups (P<0.05). The lowest weight of the spleen was seen in the maghemite 500 group, which had significant contrast compared to the maghemite 100 group (P<0.05) (
Figure 2A).
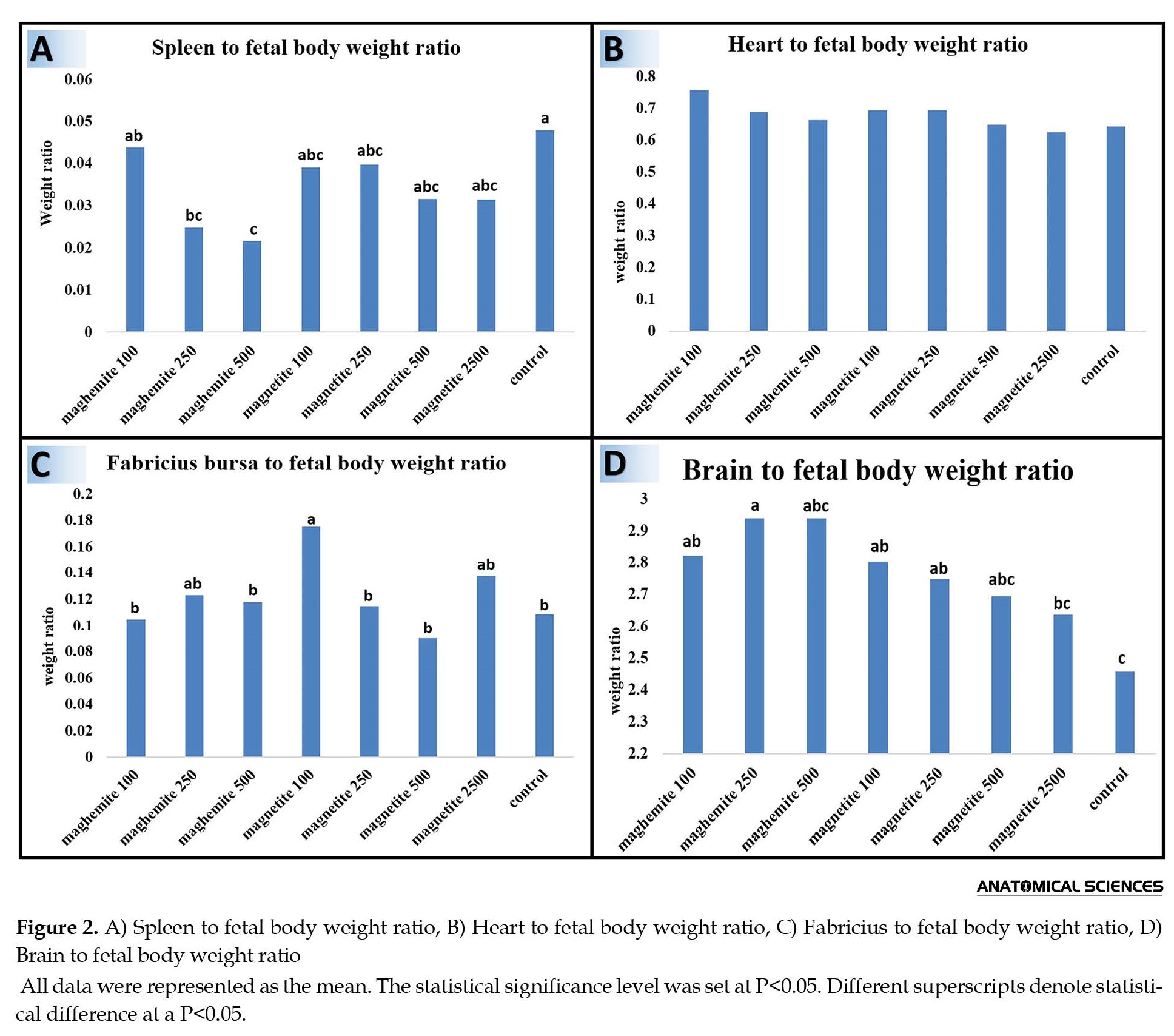
The highest heart-to-fetal body weight ratio was observed in the maghemite 100 group and the lowest one was for magnetite 2500. However, there was not any significant difference between all groups in terms of heart-to-fetal body weight ratio (P>0.05) (
Figure 2B).
The bursa Fabricius to fetal body weight ratio assessment showed that the highest rate belonged to the maghemite 100 group, which had a significant difference in comparison with all groups except magnetite 2500 and maghemite 250 (P<0.05) (
Figure 2C).
The brain-to-fetal body weight ratio was lowest in the control group, which had a significant difference compared to the magnetite 100 and 250 and maghemite 100 and 250 groups (P<0.05). The highest level of the mentioned ratio was detected in the maghemite 250 group, which had a significant difference compared to the control and magnetite 2500 groups (P<0.05) (
Figure 2D).
Histological findings
In all maghemite and magnetite groups, edema, necrosis, and vacuolation of neurons were distinct (
Figures 3 and
4).
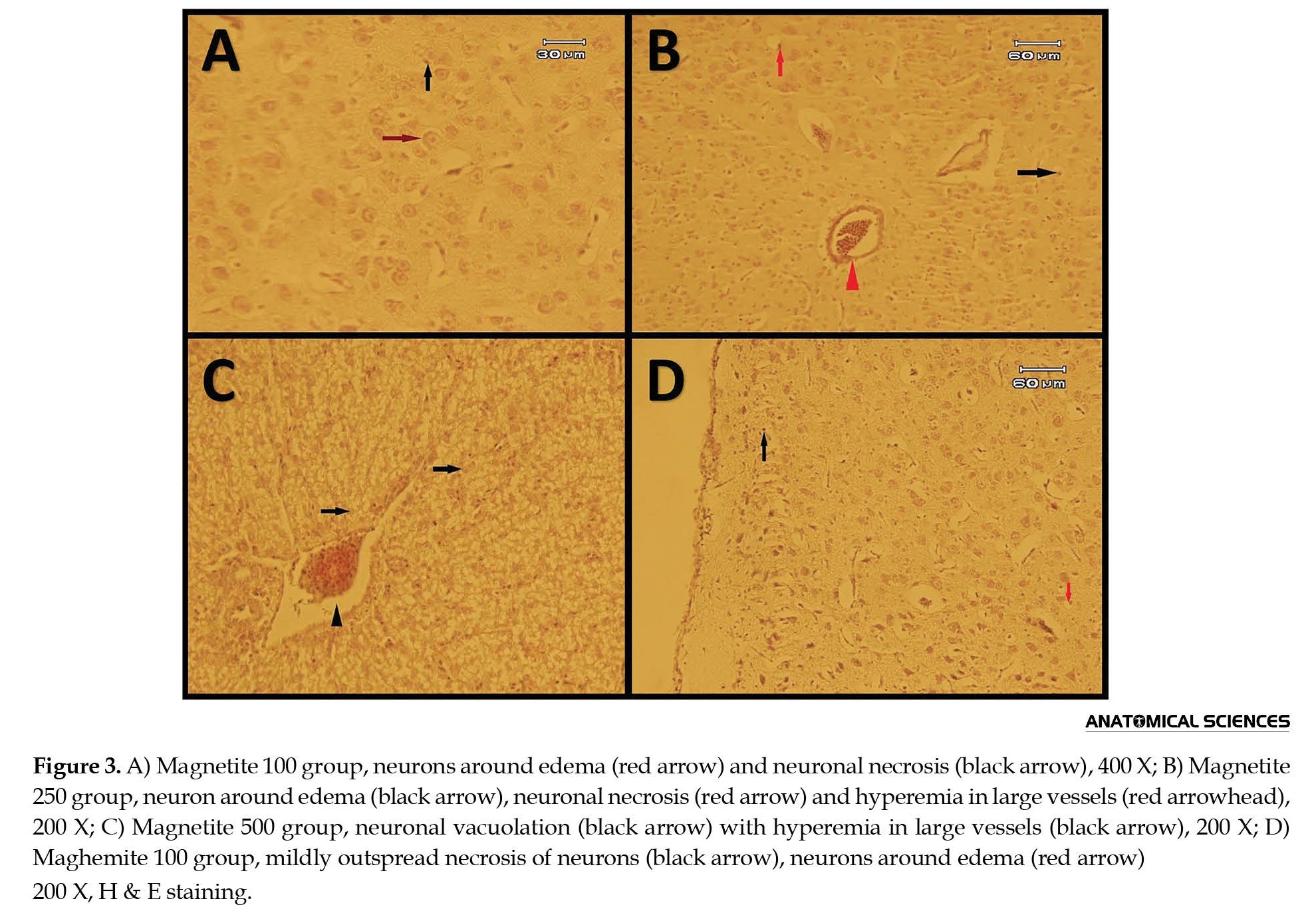
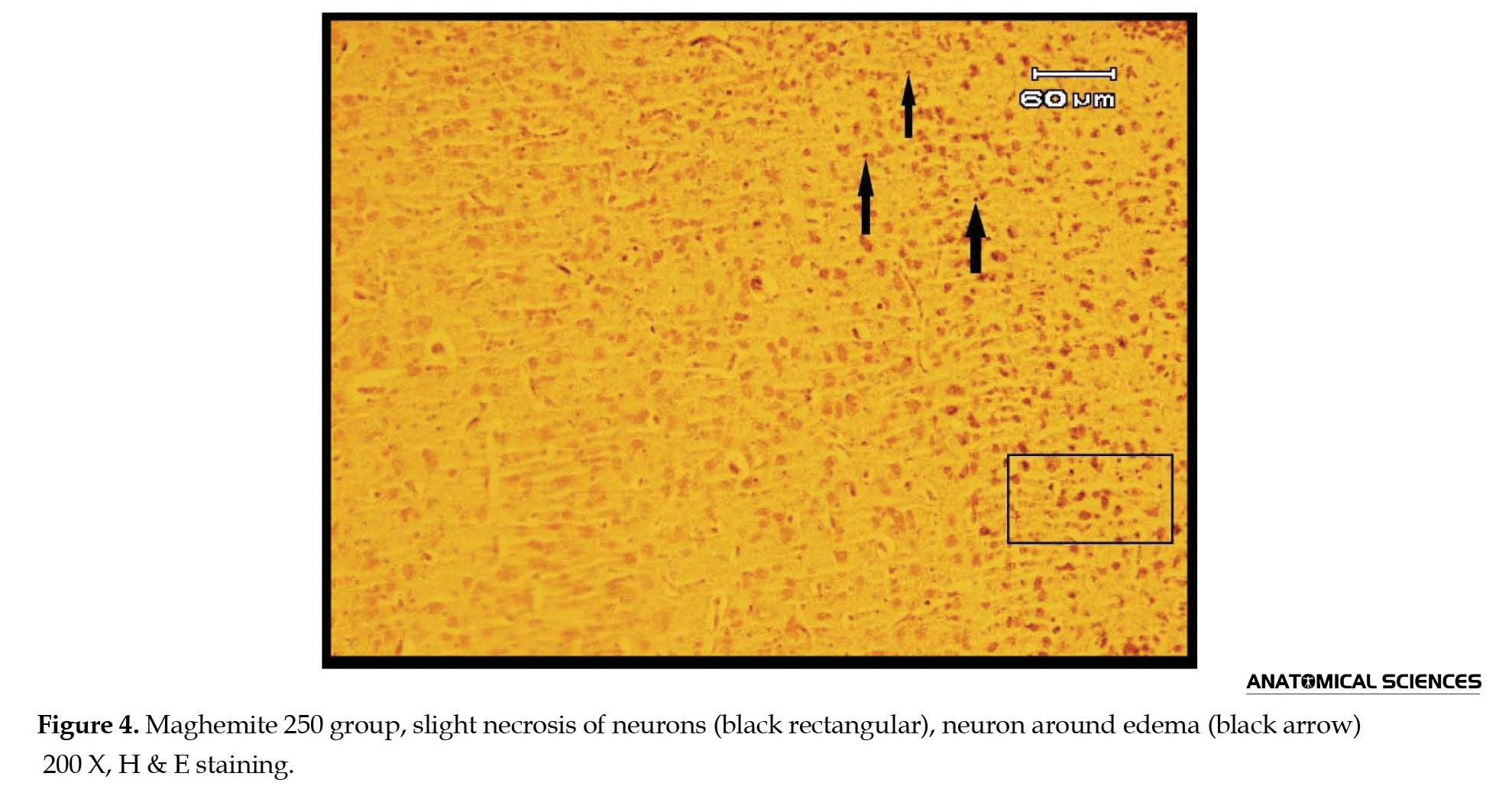
Hyperemia was distinguished in magnetite 250, 500, and 2500 and maghemite 100 and 250 groups (
Figures 3B,
3C,
3D,
4 and
6).
In groups, magnetite 250 and 2500 and maghemite 250, centered chromatolysis was recognizable (
Figures 3B,
4, and
6). Neuronophagia was seen in magnetite 250 and 500 and maghemite 100 and 250 groups (
Figures 3B,
3C,
3D, and
4). Also, edema around vessels was detectible in magnetite 500 and maghemite 100 groups (
Figures 3C and
3D). Satellitosis was recognized in maghemite 250 and 500 groups (
Figures 4 and
5).
Additionally, maghemite 100 and 250 groups showed the probability of apoptosis (
Figures 3D and
4).
Macroscopical findings of teratogenous samples
Teratogenous samples in macroscopy and anatomical investigations had 3 types of explicit defects: A) Lack of formation of abdominal wall muscles (
Figure 7A and
8),
B) Exposing the heart out of the thorax (
Figure 7B and
8),
C) Wings and legs had grown disproportionately and had anatomical deformities (
Figure 7C).
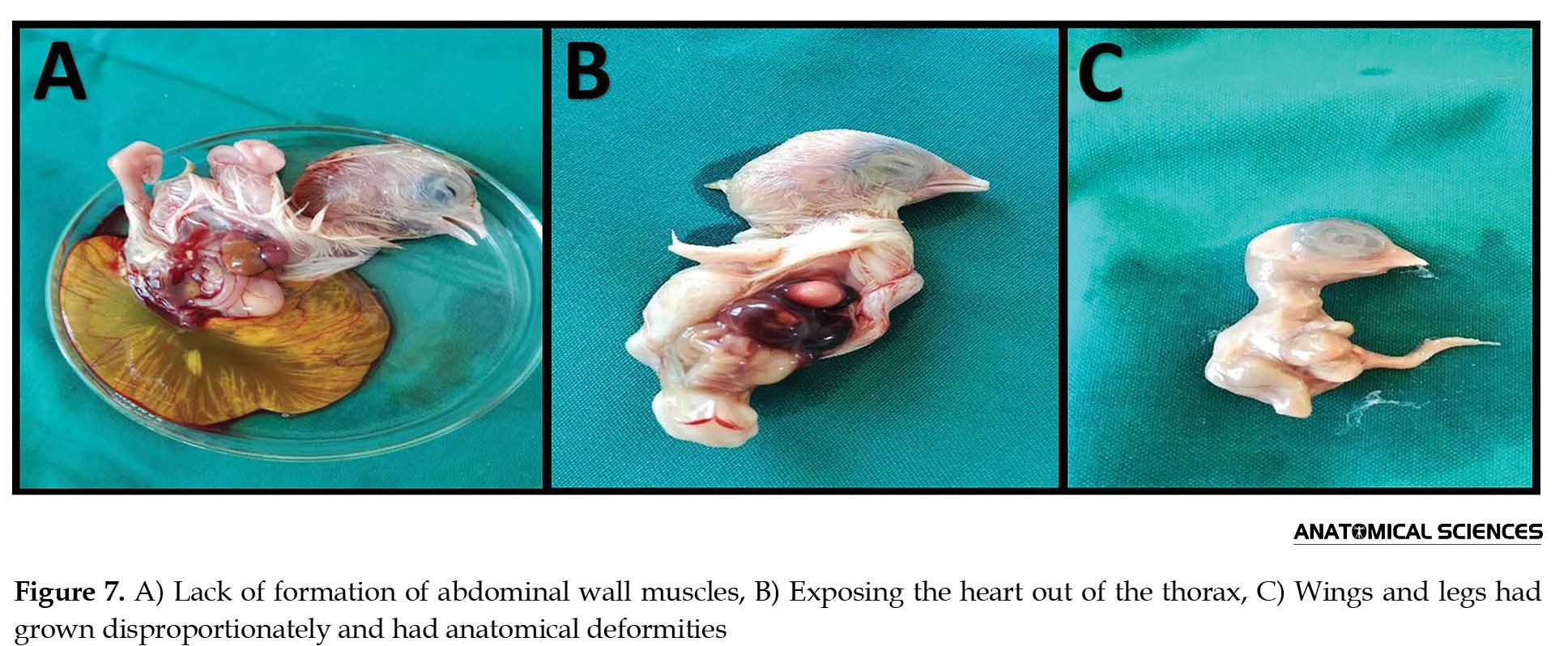
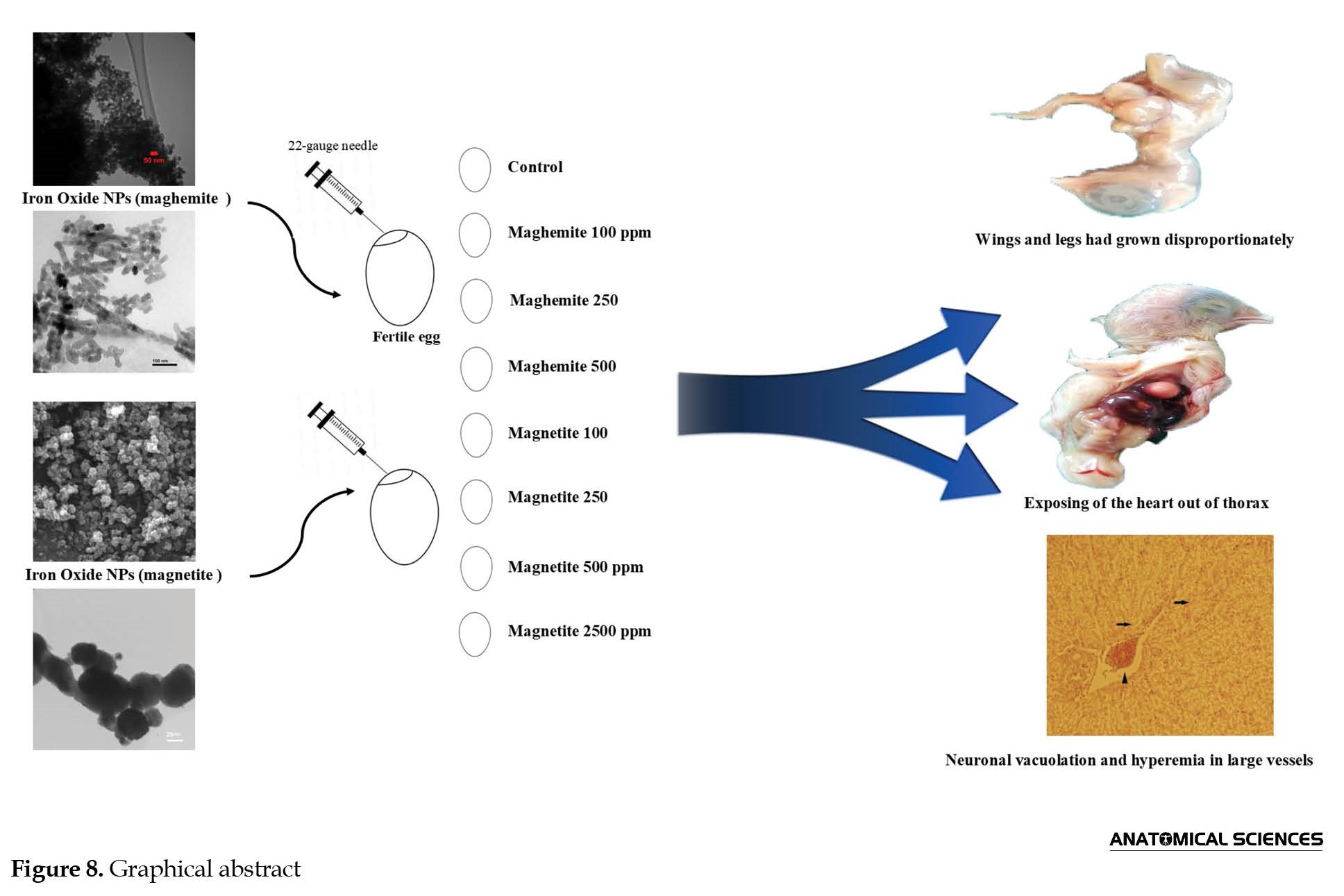
4. Discussion
In this study, iron oxide NPs such as maghemite and magnetite cause alterations in brain tissue and some abnormality in the embryo. Also, iron oxide NPs made considerable changes in the rate of some internal organs to total body weight. Among many known nanomaterials, magnetic nanoparticles have a special place and are highly regarded. Magnetic materials respond to the magnetic field; however, the relative toxicity is a major concern in applying magnetic nanoparticles. Nanomagnetic’s toxicity is related to dose, chemical composition, size, structure, solubility, surface chemistry, biological analysis, etc. [
30].
The low toxicity of iron oxide NPs, especially magnetite, and their ability to cross the blood-brain barrier (BBB) make them the appropriate choice as a Nano platform for treating malignant brain tumors. Also, the unique magnetic characteristics of magnetites can be usable along with a safe magnetic field to produce topical hyperthermia for thermotherapy and magnetic targeting of malignant brain tumors [
31].
Morphologically, iron oxide NPs include a nucleus and shell. Iron oxide (usually magnetite or maghemite), which makes the nucleus and the shell, contains an environmentally friendly material such as polysaccharide, synthetic polymer, lipid, or protein [
32]. For the application of iron oxide NPs in imaging and treatment, we need a particular surface coating which is non-toxic and biological and obtains targeted delivery with particle mechanized in a specific area [
7]. The final hydrodynamic size of iron oxide NPs plays an important role in their application because it may be difficult to target malignant brain tumors with nanoparticles larger than 100 nm.
Exposing cells to NPs may cause intercellular adhesion changes, affecting cell characteristics like morphology, cellular skeleton, separation, and even cell survival. For example, magnetic iron oxide NPs without surface modification (with 50 nm diameter approximately) reduced the cellular adhesion by 64% compared to iron oxide NPs coated by polyethylene glycol (PEG). The functional metabolism of NPs and the function of magnetic NPs with different coatings are various. Uncoated NPs are more toxic than coated NPs; however, the type of coatings affects toxicity. For example, NPs coated with polyethylene glycol or albumin have lower toxicity than those coated with dextran [
33].
Although in vitro measurement of magnetic nanoparticles toxicity on various cells confirms basic information on the safety of these nanoparticles, these tests do not provide the inside of the body. Also, it can be mentioned that in vitro cellular toxicity may be higher than in vivo, because, in cell cultures, NPs and their destroyed products which affect cell viability, remain in close contact with the cells and show continuous effects of toxicity [
34].
Prasek et al. [
35] investigated the effects of platinum NPs on chicken embryo growth and brain morphology and showed that these NPs had no effects on embryo growth but caused a decrease in the number of proliferative cells in the brain tissue. The results of this experimental study verify them. Also, in this research, iron oxide NP had no effects on embryo growth in experimental groups and revealed edema around neurons and necrosis in the brain, and in terms of weight, experimental groups had an increase in comparison with the control group.
Pineda et al. [
36] evaluated copper NPs’ effects on chicken embryo metabolism over several days and displayed that oxygen consumption in experimental groups decreased remarkably compared with the control group. Also, the weight of internal organs such as the liver, heart, and intestine was lower in the experimental groups. However, the concentration level of immunoglobulins and gene expression did not change. On the contrary, the present study revealed that the lowest liver weight in the control group and the heart-to-fetal body ratio did not show significant differences between groups.
Taze et al. [
37] studied the effects of iron oxide NPs and iron oxide NPs coated with zeolite on the Mediterranean mussel (Mytilus galloprovincialis) and recorded physiological changes caused by oxidative stress in hemocytes of treated mussels in comparison with the control group. Finally, they revealed that using NPs in medicine and the environment requires additional research to confirm the safety of these nanoparticles. According to the recorded toxicity and teratogenicity of iron oxide NPs in the present study, to use these NPs, further studies with low doses should be carried out to determine the safety margin of iron oxide NPs.
Abdolmaleki and Zahri [
38] investigated the teratogenic and cellular toxicity effects of the Salen Vanadium Oxide (VOS) complex on the chicken embryo, hepatocytes, and fibroblasts. They reported that in low concentration levels of the VOS complex did not have any remarkable toxic effects on the embryo but extraordinarily affected the cell proliferation, which antiproliferative effects of this complex on fibroblastic cells were more than hepatocytes. In contrast with the above study, in this research, low concentration levels like high concentration levels made teratogenic effects, which most likely can be caused by the very small size of iron oxide NPs that even at low concentration levels can be effective due to easily crossing the biological barrier of animal cells.
Zhao et al. [
39] said that iron oxide NPs have caused changes in the internal structure of amino acids. Also, they noted that iron oxide NPs and their hydrates have low toxicity. Changes in amino acid’s internal structure can be a reason for toxicity and teratogenicity in this study. Also, in this study, like Zhao et al., the toxicity of iron oxide NPs was proved, which appeared in the brain as edema around neurons, neuronophagia, etc.
Nori et al. [
40] investigated the short-term effects of iron oxide NPs on the liver and kidney of rats and revealed that short-time consumption of iron oxide NPs made no certain toxicity in the body. These consequences are contrary to the results of the present experimental study on the liver, which can be attributed to the difference in the time of iron oxide NPs consumption or higher liver capacity of rats against iron oxide NPs toxicity compared with chicken embryo liver.
Hajirahimi et al. [
41] showed that iron oxide and zinc NPs had adverse tissue effects on the liver of living organisms even at low levels but improved growth indices of rainbow trout. This study verifies the toxic impact of iron oxide NP in the liver as present investigation. But, in the present study, improved growth indices were not observed, which can be related to the difference in growth between embryo and infant.
The toxic effects of iron oxide NPs coated with hydro extract of brown algae on ovarian cancer cells were investigated by Namvar et al. [
42]. This study showed that brown algae extract was an appropriate alternative for stabilizing and resuscitating colloid solution of iron oxide NPs, which induces an apoptotic effect on the A2780cp cell line and makes these NPs a relevant therapeutic option for ovarian cancer. In the current evaluation, the apoptotic effects of iron oxide NPs in brain tissue appeared in maghemite 100 and 250 groups. According to Namvar et al. [
42] study, iron oxide NPs can be used for inducing apoptosis in tumor cells.
In conclusion, nanoparticle usage has disparate consequences. Because of their tiny size, they can cross through membranes and affect cells. Therefore, finding safe doses of nanoparticles needs more investigation. In this experimental study, iron oxide NPs affect the growth and development of embryos and can cross the BBB and penetrate the brain tissue, affecting brain morphology.
Compliance with ethical guidelines
All ethical principles are considered in this article. The participants were informed of the purpose of the research and its implementation stages. They were also assured about the confidentiality of their information and were free to leave the study whenever they wished, and if desired, the research results would be available to them. A written consent has been obtained from the subjects. Principles of the Helsinki Convention was also observed.
Funding
This research was supported by the research project (No. 1679), funded by the University of Tabriz.
Authors' contributions
Conceptualization and supervision: Ghasem Akbari and Mehdi Basaki; Methodology: Marzieh Hejazi and Heresh Rezaei; Investigation, Data collection: Heresh Rezaei and Mohammad Babaei; Data analysis: Mohammad Babaei; Funding acquisition and resources: Ghasem Akbari; Writing the paper: Mohammad Babaei and Ghasem Akbari.
Conflict of interest
The authors declared no conflict of interest.
Acknowledgments
The authors would like to thank University of Tabriz for financial support and Ali Kalantari-Hesari for final review of English grammar.
References
- Nadar A, Banerjee AM, Pai MR, Meena SS, Patra AK, Sastry PU, et al. Immobilization of crystalline Fe2O3 nanoparticles over SiO2 for creating an active and stable catalyst: A demand for high-temperature sulfuric acid decomposition. Applied Catalysis B: Environmental. 2021; 283:119610. [DOI:10.1016/j.apcatb.2020.119610]
- Kastrinaki G, Lorentzou S, Karagiannakis G, Rattenbury M, Woodhead J, Konstandopoulos AG. Parametric synthesis study of iron-based nanoparticles via aerosol spray pyrolysis route. Journal of Aerosol Science. 2018; 115:96-107. [DOI:10.1016/j.jaerosci.2017.10.005]
- Mohajerani A, Burnett L, Smith JV, Kurmus H, Milas J, Arulrajah A, et al. Nanoparticles in construction materials and other applications, and implications of nanoparticle use. Materials. 2019; 12(19):3052. [DOI:10.3390/ma12193052] [PMID]
- Sidiropoulou E, Feidantsis K, Kalogiannis S, Gallios GP, Kastrinaki G, Papaioannou E, et al. Insights into the toxicity of iron oxide nanoparticles in land snails. Comparative Biochemistry and Physiology. Toxicology & Pharmacology . 2018; 206-207:1-10. [DOI:10.1016/j.cbpc.2018.02.001] [PMID]
- Mandilas C, Daskalos E, Karagiannakis G, Konstandopoulos AG. Synthesis of aluminum nanoparticles by arc plasma spray under atmospheric pressure. Materials Science and Engineering: B. 2013; 178(1):22-30. [DOI:10.1016/j.mseb.2012.10.004]
- Shasha C, Krishnan KM. Nonequilibrium dynamics of magnetic nanoparticles with applications in biomedicine. Advanced Materials. 2021; 33(23):e1904131. [DOI:10.1002/adma.201904131] [PMID]
- Malhotra N, Lee JS, Liman RAD, Ruallo JMS, Villaflores OB, Ger TR, et al. Potential toxicity of iron oxide magnetic nanoparticles: A review. Molecules. 2020; 25(14):3159. [DOI:10.3390/molecules25143159] [PMID]
- Aragaw TA, Bogale FM, Aragaw BA. Iron-based nanoparticles in wastewater treatment: A review on synthesis methods, applications, and removal mechanisms. Journal of Saudi Chemical Society. 2021; 25(8):101280. [DOI:10.1016/j.jscs.2021.101280]
- Laurent S, Saei AA, Behzadi S, Panahifar A, Mahmoudi M. Superparamagnetic iron oxide nanoparticles for delivery of therapeutic agents: Opportunities and challenges. Expert Opinion on Drug Delivery. 2014; 11(9):1449-70. [DOI:10.1517/17425247.2014.924501] [PMID]
- Peng H, Guo J. Removal of chromium from wastewater by membrane filtration, chemical precipitation, ion exchange, adsorption electrocoagulation, electrochemical reduction, electrodialysis, electrodeionization, photocatalysis and nanotechnology: A review. Environmental Chemistry Letters. 2020; 18(6):2055-68. [DOI:10.1007/s10311-020-01058-x]
- Rathi BS, Kumar PS. A review on sources, identification and treatment strategies for the removal of toxic Arsenic from water system. Journal of Hazardous Materials. 2021; 418:126299. [DOI:10.1016/j.jhazmat.2021.126299] [PMID]
- Thabet RH, Fouad MK, Ali IA, El Sherbiney SA, Tony MA. Magnetite-based nanoparticles as an efficient hybrid heterogeneous adsorption/oxidation process for reactive textile dye removal from wastewater matrix. International Journal of Environmental Analytical Chemistry. 2023; 103(11):2636-58. [DOI:10.1080/03067319.2021.1896716]
- Misaelides P. Application of natural zeolites in environmental remediation: A short review. Microporous and Mesoporous Materials. 2011; 144(1-3):15-8. [DOI:10.1016/j.micromeso.2011.03.024]
- Tica D, Udovic M, Lestan D. Immobilization of potentially toxic metals using different soil amendments. Chemosphere. 2011; 85(4):577-83. [DOI:10.1016/j.chemosphere.2011.06.085] [PMID]
- Salem Attia TM, Hu XL, Yin DQ. Synthesized magnetic nanoparticles coated zeolite for the adsorption of pharmaceutical compounds from aqueous solution using batch and column studies. Chemosphere. 2013; 93(9):2076-85. [DOI:10.1016/j.chemosphere.2013.07.046] [PMID]
- McCarthy JR, Weissleder R. Multifunctional magnetic nanoparticles for targeted imaging and therapy. Advanced Drug Delivery Reviews. 2008; 60(11):1241-51. [DOI:10.1016/j.addr.2008.03.014] [PMID]
- Zhao CY, Cheng R, Yang Z, Tian ZM. Nanotechnology for cancer therapy based on chemotherapy. Molecules. 2018; 23(4):826. [DOI:10.3390/molecules23040826] [PMID]
- Tang C, He Z, Liu H, Xu Y, Huang H, Yang G, et al. Application of magnetic nanoparticles in nucleic acid detection. Journal of Nanobiotechnology. 2020; 18(1):62. [DOI:10.1186/s12951-020-00613-6] [PMID]
- Cardoso VF, Francesko A, Ribeiro C, Bañobre-López M, Martins P, Lanceros-Mendez S. Advances in magnetic nanoparticles for biomedical applications. Advanced Healthcare Materials. 2018; 7(5):1700845. [DOI:10.1002/adhm.201700845] [PMID]
- Zhi D, Yang T, Yang J, Fu S, Zhang S. Targeting strategies for superparamagnetic iron oxide nanoparticles in cancer therapy. Acta Biomaterialia. 2020; 102:13-34. [ [DOI:10.1016/j.actbio.2019.11.027] [PMID]
- Wankhede M, Bouras A, Kaluzova M, Hadjipanayis CG. Magnetic nanoparticles: An emerging technology for malignant brain tumor imaging and therapy. Expert Review Of Clinical Pharmacology. 2012; 5(2):173-86. [DOI:10.1586/ecp.12.1] [PMID]
- Palanisamy S, Wang YM. Superparamagnetic iron oxide nanoparticulate system: Synthesis, targeting, drug delivery and therapy in cancer. Dalton Transactions. 2019; 48(26):9490-515. [DOI:10.1039/C9DT00459A] [PMID]
- Corot C, Robert P, Idée JM, Port M. Recent advances in iron oxide nanocrystal technology for medical imaging. Advanced Drug Delivery Reviews. 2006; 58(14):1471-504. [DOI:10.1016/j.addr.2006.09.013] [PMID]
- Lodhia J, Mandarano G, Ferris NJ, Eu P, Cowell S. Development and use of iron oxide nanoparticles (Part 1): Synthesis of iron oxide nanoparticles for MRI. Biomedical Imaging and Intervention Journal. 2010; 6(2):e12. [ [DOI:10.2349/biij.6.2.e12] [PMID]
- Thorek DL, Chen AK, Czupryna J, Tsourkas A. Superparamagnetic iron oxide nanoparticle probes for molecular imaging. Annals of Biomedical Engineering. 2006; 34(1):23-38. [DOI:10.1007/s10439-005-9002-7] [PMID]
- Patra JK, Das G, Fraceto LF, Campos EVR, Rodriguez-Torres MDP, Acosta-Torres LS, et al. Nano based drug delivery systems: Recent developments and future prospects. Journal of Nanobiotechnology. 2018; 16(1):71. [DOI:10.1186/s12951-018-0392-8] [PMID]
- Badi N, Fazelipour S, Naji T, Babaei M, Kalantari Hesari A. Histomorphometric and biochemical study of liver and thyroid hormones following administration of MoO3 nanoparticles in female rats. Iranian Journal of Veterinary Medicine. 2022; 16(2):188-201. [DOI: 10.22059/IJVM.2021.330872.1005196]
- Teuşan A, Baranov N, Dmour R, Vizitiu A, Jelihovschi I, Teuşan V. The effects of metal nanoparticles on embryos of different animal species. A review. International Journal of Medical Dentistry. 2015; 19(3):179-82. [Link]
- Sharma G, Sharma AR, Lee SS, Bhattacharya M, Nam JS, Chakraborty C. Advances in nanocarriers enabled brain targeted drug delivery across blood brain barrier. International Journal of Pharmaceutics. 2019; 559:360-72. [DOI:10.1016/j.ijpharm.2019.01.056] [PMID]
- Dadfar SM, Roemhild K, Drude NI, von Stillfried S, Knüchel R, Kiessling F, et al. Iron oxide nanoparticles: Diagnostic, therapeutic and theranostic applications. Advanced Drug Delivery Reviews. 2019; 138:302-25. [DOI:10.1016/j.addr.2019.01.005] [PMID]
- Aghebati-Maleki A, Dolati S, Ahmadi M, Baghbanzhadeh A, Asadi M, Fotouhi A, et al. Nanoparticles and cancer therapy: Perspectives for application of nanoparticles in the treatment of cancers. Journal of Cellular Physiology. 2020; 235(3):1962-72. [DOI:10.1002/jcp.29126] [PMID]
- Zhao S, Yu X, Qian Y, Chen W, Shen J. Multifunctional magnetic iron oxide nanoparticles: An advanced platform for cancer theranostics. Theranostics. 2020; 10(14):6278-309. [DOI:10.7150/thno.42564] [PMID]
- Hadjipanayis CG, Machaidze R, Kaluzova M, Wang L, Schuette AJ, Chen H, et al. EGFRvIII antibody-conjugated iron oxide nanoparticles for magnetic resonance imaging-guided convection-enhanced delivery and targeted therapy of glioblastoma. Cancer Research. 2010; 70(15):6303-12. [DOI:10.1158/0008-5472.CAN-10-1022] [PMID]
- Spirou SV, Costa Lima SA, Bouziotis P, Vranješ-Djurić S, Efthimiadou EΚ, Laurenzana A, et al. Recommendations for in vitro and in vivo testing of magnetic nanoparticle hyperthermia combined with radiation therapy. Nanomaterials. 2018; 8(5):306. [DOI:10.3390/nano8050306] [PMID]
- Prasek M, Sawosz E, Jaworski S, Grodzik M, Ostaszewska T, Kamaszewski M, et al. Influence of nanoparticles of platinum on chicken embryo development and brain morphology. Nanoscale Research Letters. 2013; ;8(1):251. [DOI:10.1186/1556-276X-8-251] [PMID]
- Pineda L, Sawosz E, Vadalasetty KP, Chwalibog A. Effect of copper nanoparticles on metabolic rate and development of chicken embryos. Animal Feed Science and Technology. 2013; 186(1-2):125-9. [DOI:10.1016/j.anifeedsci.2013.08.012]
- Taze C, Panetas I, Kalogiannis S, Feidantsis K, Gallios GP, Kastrinaki G, et al. Toxicity assessment and comparison between two types of iron oxide nanoparticles in Mytilus galloprovincialis. Aquatic Toxicology. 2016; 172:9-20. [DOI:10.1016/j.aquatox.2015.12.013] [PMID]
- Abdolmaleki A, Zahri S. Comparison of toxicity and teratogenic effects of salen and vo-salen on chicken embryo. Drug and Chemical Toxicology. 2016; 39(3):344-9. [DOI:10.3109/01480545.2015.1121492] [PMID]
- Zhao B, Wang Y, Guo H, Wang J, He Y, Jiao Z, et al. Iron oxide (III) nanoparticles fabricated by electron beam irradiation method. Materials Science Poland. 2007; 25(4):1143-8. [Link]
- Nori A, Amiri GH, Taj B, Nasreesfahani M, Taj S, Valiyani A. [The effect of magnetic iron oxide nanoparticles on mice liver and kidney (Persian)]. Journal of Kerman University of Medical Sciences. 2012; 19(3):243-53. [Link]
- Hajirahimi A, Farokhi F, Tokmehchi A. [Effects of Iron oxide and zinc nanoparticles on the liver and muscles in rainbow trout (Oncorhynchus mykiss) (Persian)]. Journal of Animal Research (Iranian Journal of Biology). 2015; 28(3):293-306. [Link]
- Namvar F, Amini E, Mahdavi M. [Cytotoxic effect of magnetic iron oxide nanoparticles coated with seaweed aqueous extract against ovarian cancer cells (Persian)]. KAUMS Journal (FEYZ). 2015; 19(2):94-101. [Link]